9 Ecological Engineering
The last topic in the habitat-focused techniques group centers on the idea of ecological engineering. Ecological engineering is different from the respiration because it is focused on designing and reconstructing environments consistent with ecological principles, but it also attempts to integrate the needs of human society within its natural environment. We will explore the background of ecological engineering within a framework of ecological stress and health, and delve into the ideas that set ecological engineering apart from restoration. We will end with a case study on the Chicago waterfront ecological engineering project.
A SHIFT IN THINKING FROM RESTORATION TO ECOLOGICAL ENGINEERING
Human domination of the earth has become widespread and pervasive (Vitousek et al. 1997). Alteration of ecosystems by direct changes such as land cover replacement, introduction of new species, pollution, and development has resulted in new ecosystems unlike those in their original state (Ellis 2011). We have come to recognize that indirect human-motivated changes such as climate change, water use and rerouting, and the decline of biodiversity are changing the natural world (Braun 2020). Generally, these direct and indirect influences of humans are creating new ecosystems that are novel in properties, possess new biological communities, and support people. Much of earth is already a merger of natural and human processes, and we must understand and manage these novel ecosystems for both natural and human benefits (Hobbs et al. 2006). These new ecosystems have to be understood and often recreated from damaged and abandoned lands and waters.
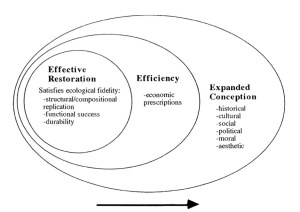
Restoration has been the typical method used in the past to improve degraded ecosystems. Restoration applies a static approach to dynamic ecosystems to shift the site to a fixed state (e.g., an ecosystem or habitat that once existed in the past). The field of restoration has received criticism from within and beyond the professional restoration community as being impractical because of irreplaceable losses, irreversible changes, and for being backward looking. Further, restoration often progresses on an ad hoc, site- and situation-specific basis and proceeds with considerable subjectivity in determining restoration goals. Goals are often idealistic and not feasible under prevailing economic, social, and political circumstances. Restoration is viewed as an “ art ” rather than a science and often relies upon intuition rather than a documented knowledge base (Choi et al. 2008).
More recently there has been a shift away from site- and situation-specific restoration projects to a broader approach (Hobbs 2004). The shift (Figure 9.1) has been toward forward-looking restoration practices, acknowledging a changing and unpredictable environment, assuming ecological communities are dynamic in nature, connecting to landscape elements, and seeking public support and community participation in setting realistic goals (Choi et al. 2008). The new processes driving ecological engineering build on direct links to human interests for support from people, and are economically, ethically, socially, and politically acceptable, and well-justified in these terms (Choi 2004; Choi et al. 2008).
Ecological engineering has been going on for years under specialties titled landscape rehabilitation, nature engineering, renaturing, ecotechnology, biomanipulation, ecological restoration, reconciliation ecology, designer ecosystems, invented nature, and artificial ecology. In general, natural-human ecosystems have not been studied much, although there is now a strong trend in this direction.
There are common elements in ecological engineering such as a move away from the thinking that people and nature are incompatible. Instead, human interests and ecological benefits are used to define objectives for restoring a site to meet cultural and ecological interests. Social and aesthetic interests often influence the design objectives through community participation in planning. One common goal is to restore healthy relationships between residents and natural spaces by creating harmony between human and ecological activities. The kinds of features that are often restored are initially raised by interested stakeholders. Aesthetic qualities are important in strengthening public support for maintaining the restoration. A good ecosystem plan may rely on providing natural services for human activities, and maintaining desired properties in support of societal values.
The process of ecological engineering helps to set realistic objectives that address ecological, economic, and social benefits where copying attributes of a natural system has little relevance. Creative practices are especially needed in highly stressed ecosystems that are not expected to return to a near- natural state. Plans commonly consider how the ecosystem will function and persist in the near-term and long-term future. Merging ecological and social considerations with engineering practices differentiates this approach from past restoration approaches with respect to damaged or abandoned environmental settings.
DEFINITION AND HISTORICAL BASIS FOR ECOLOGICAL ENGINEERING

Ecological engineering has been defined in various ways but the core concepts involve designing and reconstructing environments for nature and people (Mitsch and Jørgensen 1989; Jackson et al. 1995; Mitsch and Jørgensen 2003; Odum and Odum 2003). The sustainability of these novel environments is also a priority (Costanza 2012). The goal is to achieve persistent ecosystems that serve the needs of both people and nature in an efficient manner. The most common definition of ecological engineering was provided by Mitsch (1996) as the design of sustainable systems, consistent with ecological principles, which integrate human society with its natural environment for the benefit of both. The key elements of the definition are that engineering practices need to be grounded in ecological science, focused on human interactions with the natural ecosystem, designed for both people and nature, and take into account both human and natural values (Figure 9.2). Overall, ecological engineering has grown in importance in environmental management because of the increasing need to address human welfare and the natural environment in settings that are damaged or dominated by humans (Kareiva et al. 2007).
This relatively new approach to the practice of environmental management was introduced in the United States during the 1960s by Howard T. Odum and in China by ecologist Ma Shijun. Both scientists based their original ideas on maintaining ecosystem dynamics and cycling of energy and substances. Twenty years later these ideas also emerged in Europe as a technological approach for ecosystem management with a strong basis in ecological understanding. This field is a good fit for both ecologists and engineers that are designing and constructing ecosystems in human-dominated settings, such as natural resource specialists, environmental and civil engineers, agroecologists, and landscape planners. The field also provides ample material for scientists and managers who are striving to restore environments that have been substantially degraded by human activities, and environments that must blend human and ecological values.
FUNDAMENTAL ECOLOGICAL ENGINEERING PRINCIPLES
Research aimed at ecological engineering has been less developed than other techniques used for environmental management and has been scattered across different fields. Ecologists tend to focus their research on natural or wild ecosystems that can provide information on the dynamics of nature. Some applied sciences such as forestry, agriculture, and landscape planning focus on the control and maintenance of productive ecosystems for direct human benefits. In the middle are ecosystems that are neither completely natural nor entirely controlled for commodity production. These are the types of ecosystems that are of interest in ecological engineering. These are ecosystems that may have been damaged so much that they can no longer revert to a natural state, become self-organized into an ecologically desirable condition, or be used for human commodity production without substantial efforts. Other ecosystems that are of interest to ecological engineering are those that have the capacity to support natural communities with mitigation activity, highly managed systems for waste treatment, abandoned lands and waters, newly constructed habitats such as wetlands, and environments constructed for housing people which still retain some natural attributes. The science aimed at studying these ecosystems tends to focus on ecosystem stress and environmental health, independent of natural conditions. Concepts that can define ecosystem quality are resilience, resistance to change, self-organization capacity, and a -diverse structure. There is a need for a framework that defines the successful creation and improvement of ecosystems that provide persistent benefits for both nature and people. This has not been accomplished yet but researchers’ interest in pursuing this vision is now growing.
The incorporation of ecological principles into ecosystem engineering is what distinguishes this environmental management technique from standard engineering practices (Example in Figure 9.3). Engineering designs traditionally aim for a static end product that will provide benefits to people and maintain operations. Ecosystems are dynamic, meaning they vary through time, but persist within a range that defines system behaviors. One principle of ecological engineering is shifting from classic engineering designs to a looser ecological design that recognizes a flexible functional space (Bergen et al. 2001). That is, ecological engineering has to acknowledge that ecosystems do need space to vary, but not so much that the ecosystem transitions to a new stable status. Wide tolerances and multiple natural components have to be specified, as well as measures that keep the system’s dynamics within a range that is seen as acceptable. Redundancy in ecosystem structures (e.g., food chains) is one well recognized way to provide built-in resilience to stressors (Costanza and Mageau 1999). In traditional engineering, redundancy is not seen as needed. Thus, the specifications required for ecosystem engineering have to: include varying community composition, ensure that tolerant or undesirable species do not dominate the landscape, provide space and benefits for people, and incorporate redundant natural components for security.
Another ecological principle that should be followed is to design and manage projects with efficiency, low-energy inputs, and sustainability in mind. Ecosystems are like any other system which has internal components that interact to shape properties and self-organize. Relying on ecosystem self-organization leads to sustainability, because an emergent system is more likely to remain in an approximate configuration. By contrast, high energy inputs can certainly be used to maintain ecosystem properties, but they are energy intensive, use regular stocking of species, require heavy landscape maintenance, involve retention and diversion of water, utilize extensive construction, and other strategies. Working with natural processes to maintain approximate ecosystem conditions can be less energy intensive, less costly, and promote sustainability in the long-term. This strategy differs from standard engineering practices and calls for a shift in thinking in terms of how natural processes can be used to create and maintain a desired ecological state.
A final principle based on the science of ecosystems and their maintenance focuses on place. Designs should be very context sensitive. Specialized species and habitat-linked communities need full consideration. The physical constraints on what could persist in the ecosystem need attention. Culture, aesthetics, and human values are equally important because local people will be part of the ecosystem and internally shape the outcome through time. Public support and natural constraints are associated with the local context and define what can be achieved and maintained in an ecosystem with diverse benefits.
One criticism of the ecological engineering field is that the strategy or evaluation steps are rarely published to convey lessons for planning future ecological engineering projects. These trends cause this environmental management technique to be poorly documented and to omit common practices. There is a need to learn from past cases, and develop principles for future practices.
ECOSYSTEM STRESS
Stress on ecosystems is an external force that disrupts organization, processes, and functions (Rapport et al. 1985). The effects are internal to the ecosystem but are a response to an external pressure. While not all stresses elicit the same responses, common symptoms have been recognized as attributes of degraded ecosystems. Energetics of ecosystems tend to be balanced with the rate of production and respiration. Under stress, respiration rates commonly increase, and the rate of both production and respiration often increase relative to biomass of plants and animals. Further, energy inputs to the ecosystem become more important, and export of primary production tends to increase. Overall, this is a pattern of less efficient use of internal energy in an ecosystem, and an increased loss of energy beyond the ecosystem. Nutrient cycling shows a similar pattern, where nutrient turnover increases, cycling is disrupted, and nutrient loss increases. Under ecosystem stress, biological community structure changes with a trend toward decreasing organism size, shortening life cycles, and increasing r-strategists (organisms that are opportunistic, fast reproducing, and have a high capacity for population increase). Consequently, food chains simplify, species diversity tends to go down, and dominance of tolerant taxa increases (Schindler et al. 1985). Overall stress on ecosystems commonly simplifies ecosystem structure and functions, increasing the loss of energy and nutrients that power the system. Frequently stressed ecosystems appear in the early stages of building complex biological communities ,with weaker interactions among species, less organized communities, and less diverse flora and fauna. These attributes can mark an ecosystem as in poor condition and in need of mitigation measures to reverse these trends.
Table 9.1: Ecosystem properties expected when normal and stressed. Source:Modified from Pratt 1990
Drawing from representative empirical studies about the impacts of stress on the structure and function of ecosystems, Rapport et al. (1985), Odum (1985), Schindler (1987), Schaeffer et al. (1988), Pratt (1990), and Davies and Jackson (2006) identify a number of common stages of ecosystem responses to stress (Table 9.1). The first signs of ecosystem disruption are often seen as a reduction or loss of long- lived, large species and dominance by short-lived and opportunistic species. These species are most susceptible to habitat loss and fragmentation, prey reductions, and the accumulation of toxins. Additionally, there will be a marked change in community size structure and a loss of sensitive and specialized species. Second, the ecosystem will experience changes in primary productivity linked to nutrient availability. Afterward, redistribution of abundance and biomass will occur in some groups of taxa linked to altered habitats, and species diversity tends to be reduced. Often, tolerant and generalist species increase at the expense of sensitive and specialized species. In the end, the ecosystem will break down and transition to a new system with different properties. These signs of change in ecosystem properties signal degradation of the living environment, and a likely transition to a new ecosystem state.
An analytic model of ecosystem change in response to stressors has been developed by an expert group led by Davies and Jackson (2006). Called a biological condition gradient, this model organizes changes in ecosystem structure and function to characterize the ecosystem status (Figure 9.4). The model synthesizes ecosystem properties into six phases ranging from undisturbed, or natural ecosystem conditions, to severely altered environments with major loss of ecosystem structure and function. This method builds on the characteristics of stressed ecosystems that have been long recognized but not organized into step-wise phases. The sequence of changes starts with reductions in sensitive and specialized species followed by a clear increase in tolerant and opportunistic species. As this community transitions from native and sensitive species to tolerant species, the change greatly alters community composition and leads to new food webs, species interactions, community size structure, and life cycles. These changes result in altered ecosystem functions like energy and nutrient cycling, biomass accumulation, and efficiency of resource retention. This sequence of changes in ecosystem structure and function depicts the degradation of ecosystems, and the decline of environmental quality which should be avoided.
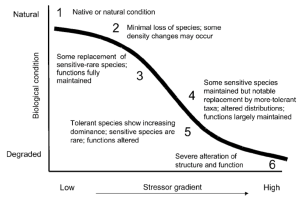
ECOSYSTEM HEALTH
With the recognition of the stress and degradation of ecosystems, ecological engineering needs to remedy such environmental distress to foster healthier landscapes. Ecosystem health is a term that is convenient because it conveys good conditions in the environment (Schaeffer et al. 1988; Rapport et al. 1998a; Rapport et al. 1998B; Costanza and Mageau 1999; Rapport and Singh 2006; Costanza 2012). The public often says they want a healthy environment, so maintaining ecosystem health is a good aim for ecosystems that are degraded and can not revert to a natural state. It is easy to make the analogy with human health when talking about ecosystems. However, this analogy is not appropriate because there is no environmental or ecosystem homeostatic process that maintains consistent internal properties in healthy ecosystems. Rather, ecosystem health refers to stress responses, damage, and altered properties and processes (Lackey 2001). Diagnosing and treating stress and damage are the central aims of ecological engineering, although undoing the undesirable ecosystem status for the benefit of nature and people is very challenging.
An ecosystem can be considered healthy when free of stress symptoms, resilient to external pressures, and sustainable (Costanza and Mageau 1999). Ecosystems are dynamic but persist within a range that lacks stress responses indicating sound status. Stable interactions between human and natural properties are also the aim of ecological engineering. A healthy status of an ecosystem includes a stable organization among both human and natural components, very active productivity, and persistence with occasional external stresses. Health can be judged based on ecosystem characteristics, behavior of the system through time, and desired services it provides to people and nature.
ECOLOGICAL ENGINEERING IN PRACTICE
Identification of public priorities requires a broad participatory process that defines objectives and prioritizes benefits. The key issue under discussion is what should be created and sustained in the conservation design. The lead organization or local government must somehow balance competing values and desires, include scientific information, and promote the benefits that are selected for a plan. Commonly in ecological engineering cases, there are no reference sites to copy, standards to be achieved, or science that defines a target ecosystem state. Therefore, a lead organization must decide which proper- ties are desired and which are unimportant, and then what can be achieved through feasible practices.
Although the public always wants a healthy environment, this is difficult to transform into clear public policy for environments that are to be newly formed and merge the interests of people with nature. Making the tradeoffs among ecological engineering alternatives and finding compromises across high and low benefit priorities is necessary and difficult. Benefits to people and nature that command top attention in an ecological engineering plan have to be formed collaboratively. The process for changing damaged properties to desirable conditions also has to be identified. Science can support these actions by helping people understand how ecosystems work, and how to assemble ecological components that will interact. An ecosystem has a dynamic nature and can be unpredictable through time. Science can portray the possible variations in conditions for public acceptance. In the end, the local public has to support a plan. Also, the engaged public has to recognize that ecological engineering aims for a broad target with natural, social, and economic aspects of developing a new ecosystem that are feasible.
Ecosystems are considered healthy by the public when they support natural resources, absorb nutrients and pollutants, and persist in a desired state despite natural perturbations. Also, it is common to design ecosystems that will not require intensive maintenance and inputs of energy, water, and cost. In short, healthy ecosystems are persistent, support high biodiversity, resist human impacts, and are productive for people and wild species (Evers et al. 2018). Also, the design of a new ecosystem for human benefits often includes sustaining agriculture, allows space for people to live and enjoy nature, resists easy overharvesting of wild species, needs little water, is not conducive to overabundant non-native species, and maintains other similar attributes. These benefits and others are expected to persist in time and be desirable for future human inhabitants. Targeted benefits should not rely on surrounding lands, people, and ecosystems. Sustainability can be designed as a product of ecosystem processes rather than energy intensive maintenance. Finally, any design is a product of societal values and ecological processes which aims for a positive change in an environmental setting (Cairns 1995).
Creating novel ecosystems can include new species for a location, new assemblages of species, different ecosystem functions, compatible human activities, but should not require continual maintenance of the setting by humans. The need for designing and engineering novel ecosystems can result from the regional extinction of species, which may require the replacement of the natural communities for persistent new assemblages of species. Interior urban settings, and intensively cultivated or permanently degraded landscapes can restrict ecological processes and recolonization by wild species of plants and animals. Finally, new ecosystems are often required for locations that have had major changes in the abiotic environment, such as mined lands, brownfields, and areas with extensive soil depletion. In these settings, engineered ecosystems are aimed at providing ecological, social, and economic benefits. Some of the common goals for establishing new ecosystems are to support greater biodiversity, integrate human activity, and provide sustainability through internal system processes. A central challenge in designing new ecosystems is to provide both human and natural services that are maintained by the novel ecosystem.
Within the conservation community there is a counter view to ecological engineering. The central concept of this view is that an ecosystem that is natural or wild is authentic, and if it has human elements in it, it is less respectable. This view hinges on the concept that “natural and wild” is one option, and “human and anthropogenic” is another option. Ecological engineering that mixes people with nature diminishes natural values. Designing ecosystems can be like art forgery, producing faked-nature as the outcome. When human design and domination of nature is attempted, then nature is destroyed. We can achieve a pleasant natural environment but that can be illusory and a false reality. These views can seem polarizing and extreme, but there is a segment of the population that promotes these ideas. They run counter to ecological engineering and there is a practical argument that must be made to improve the environment when it cannot be restored to its natural state. This debate is increasingly active as we learn more about how humans altered the earth in the past, and appreciate the worldwide scope of change humans have caused starting thousands of years ago (Martin 1973; Westphal et al. 2010).
CASE STUDY: CHICAGO WATERFRONT ECOLOGICAL ENGINEERING PROJECT
Ecological engineering is especially relevant for urban landscapes that need rehabilitation for enhanced cultural and natural benefits. Urban lands are often isolated and heavily degraded making creative planning essential to address physical, biological, and social elements (Bhattacharyya and Mahanta 2014). Experts that are park planners, landscape architects, ecological restorationists, historical preservationists, and others are often engaged. As important as diverse expert input, is broad citizen involvement is also essential to capture their own interests and perspectives. This case study illustrates this process and demonstrates challenges in restoring urban lands (Gobster and Barro 2000; Gobster 2001).
Montrose Point is a 4.5 ha (11 acres) artificial land extension into Lake Michigan made from landfill in the northern portion of Lincoln Park in Chicago (Figure 9.5). Lincoln Park itself is Chicago’s largest park and one of the largest city parks in the United States at over 485 ha (1200 acres) (Schweitz 2017). Lincoln Park is a central lakefront location with a variety of natural and developed settings which makes it an extremely popular recreation destination, with an estimated 20 million visitors annually (Gobster 2001). A plan for Montrose Point was drafted in 1938 by
Alfred Caldwell who aimed for a natural landscape design that had elements of midwest prairies, savannas, and woodlands. However, before his plan was developed the United States army took possession of Montrose Point for a radar station and Nike missile launch site to protect Chicago in the 1950s. Thus, this parcel of land is entirely non-natural and was initially used by the military. In the 1970s the Point was returned to the Chicago Park District and little happened on the Point until the 1990s when attention was focused on the entire Lincoln Park.
From the 1970s to the 1990s, the land was idle and significant growth of non-native vegetation developed. A large hedgerow of honeysuckle became established, and attracted a wide variety of birds. Bird watchers named this the “Magic Hedge” because it was common to see as many as 200 species of birds during fall and spring migrations. The Magic Hedge on Montrose Point gained national and international recognition for birding. In the late 1990s, the Chicago Park District and a non-governmental organization called the Lincoln Park Advisory Council started the Montrose Point Restoration Project to gather different visions of nature by stakeholders, design cultural and natural features for Montrose Point, and identify points of consensus and conflicting interests of stakeholders. Stakeholders included birders, environmentalists, historic preservationists, landscape architects, passive users, volleyball players, anglers, and yacht club members. A series of focus-group discussions were held and recorded to identify various interests for the design of a new Montrose Point. This process was viewed as a complex challenge linking physical, biological, and social aspects of design.
Focus-group participants overwhelmingly valued Montrose Point for its natural qualities and nature was a key element in their enjoyment of the place. There was broad agreement on improving the natural attributes of Montrose Point for plants, animals, and enjoyment by people. However, there were different views on the ways in which the Point should serve public uses, and the different user groups each had their own perspective on what was essential and important. Gobster (2001) characterized four different visions of nature on Montrose Point and summarized the perspectives based on purpose of nature (function), character of desired landscape (structure), natural and cultural significance (values), intended enjoyment (use), and symbolic features of the Point (icons) (Table 9.2). Landscape icons embodied natural and cultural features that defined what each stakeholder group strongly desired and considered essential.
Table 9.2: Summary of four visions of nature expressed by Montrose Point stakeholders.
Designed landscape – The group that identified with Alfred Caldwell’s naturalistic design for Montrose Point was largely comprised of landscape architects and historic preservationists. Their vision centered on aesthetic experiences and design as historic landscape art, and was shaped by views distinctly separate from the shoreline and city. This perspective saw an expansive meadow as an analogy to midwest prairies, multi-level vegetation surrounding the meadow as isolating walls, and a long view out to Lake Michigan. Proponents of this vision saw the short-grass meadow and long view as essential aspects that needed to be maintained. The intended uses of the Point were for passive appreciation and included relaxing, sitting, walking, and watching nature and people. The defining icons of the designed landscape perspective were the meadow and long view.
Critical habitat – This group promoted a vision of nature as habitat mainly for birds, but also other wildlife such as small mammals and butterflies. Their intent was to improve the habitat along the Lake Michigan shoreline since most of the city waterfront is constructed and heavily used by people. The main mission of their view of ecological engineering was to maintain and enhance the Magic Hedge. Many of the honeysuckle plants were reaching maximum life expectancy, thus requiring active planting of a diversity of grasses, forbs, and shrubs. This group also promoted the creation of wet areas on the Point and the idea of letting aquatic vegetation and woody debris accumulate along the lake shore. The strongest advocates of this vision were birders and they argued that Montrose Point is a special place for birds and birding. This group did not want to see meadow mowing, dog walkers, volleyball players, mountain bike riders, picnickers, anglers, sail surfers, and jet skiers. Essentially the Magic Hedge was the icon, and habitat for birds was the top priority.
Recreation – This group saw Montrose Point as a natural backdrop for recreational activities such as walking, dog walking, picnicking, biking, league volleyball, sailing, and fishing. Values that recreationists raised were: a sense of nature, isolation from the city, fresh air, and a sense of a special place. Important from a practical perspective were picnic facilities, restrooms, and parking. Different users valued additional items such as beach space for volleyball, harbor space for yachts, and the long breakwater called the “Hook.” Passive recreationists saw the stone revetment as important for lounging and enjoying the shoreline. Thus icons for recreationists varied by intended use.
Pre-European Settlement – The vision of nature for this group included the landscape attributes likely present prior to the substantial European inhabitation of Chicago. This corresponds with the common notion of a pre-Columbian baseline as the initial era of the decline of North American natural landscapes. This group advocated for creating a mosaic of prairie, savanna, and shoreline habitats with in- digenous community types, enhanced biodiversity, and native plants. In conflict with this view was the notion that Montrose Point did not exist prior to European colonization. However, this group wanted to use this sizable artificial landscape to sustain exclusively native plants and animals in close proximity to the city. Eradication of invasive and non-native species was one proposed strategy for transitioning the Point to a natural landscape. Rare and high priority conservation species could be re-established on Montrose Point such as searocket (Cakile edentula), wild mustard (Synapis arvensis), and trailing juniper (Juniperus horizontalis). Advocates wanted to focus on the restored natural landscape, and many other uses of the Point would be restricted. No icon existed on the present Montrose Point for this group, but the future icon would be the restored landscape.
The four visions of nature for Montrose Point revealed the differences in stakeholder thinking about the future of this site. Also there were differences in what should be done to enhance benefits to people and nature. The challenge was not to pick what vision was best for the Point, but to see how to integrate the perspectives and desires. The icons of three of the groups form what is needed to satisfy their desires for the future of the Point. Ultimately, the approach that was used was a hybrid vision that led to a culturally sustainable future for Montrose Point. Elements built into the resulting ecological engineering plan included Caldwell’s design of a central meadow that would be planted as a prairie, not a mowed grass field. Views would be created for aesthetic appreciation of the lake. The Magic Hedge would be expanded and maintained. Finally, a perimeter pathway around the Point would allow access for different user groups and allow people to experience the land and lake. Integration of these different visions of Montrose Point provided diverse benefits for people and nature, and was consistent with the aims of ecological engineering.
This case showed that many different ideas of “nature” exist among the public, agencies, and organizations. The successful ecological engineering plan of Montrose Point depended not on choosing the “right” nature, but instead on integrating the diverse values of stakeholders regarding culture and nature. A plan was formed that excited and encouraged historic preservationists, ecological restorationists, birders, and other recreationists to work toward restoring Montrose Point and its landscape icons as symbols of nature and culture in an urban setting. Integration is key in creating more natural places that attract the attention of people and inspire public care and admiration. The Montrose Point project is a nice example of design precipitating community involvement to create ecologically and culturally sustainable landscapes (Page 2016).
SUMMARY
As a field, ecological engineering focuses on alleviating ecosystem stress responses as these are symptoms of poor ecological health. In practice, there seems to be more emphasis on collaborative goal-setting that results in consensus on a vision for ecosystem design. The practical need to gain public support for a new ecosystem is seen as essential for long-term sustainability. The underlying goal of ecological engineering is to improve degraded and abandoned environments to provide benefits to both people and nature and this goal figures prominently in the case study. While this environmental management technique lacks an established track record and principles for success, there appears to be general consistency in many of the key features of this approach.
REFERENCES
Bergen, S.D., Bolton, S.M. and Fridley, J.L., 2001. Design principles for ecological engineering. Ecological Engineering, 18(2), pp.201-210.
Bhattacharyya, K.G. and Mahanta, M.J., 2014. Accumulation of Cd, Co, Cr, Cu, Mn, Ni, Pb and Zn in urban soil and their mobility characteristics. Advances in environmental research, 3(4), pp.321-335.
Braun, E.M., 2020. Brink of Extinction: Can We Stop Nature’s Decline? Compass Point Books. North Mankato, MN.
Cairns Jr, J., 1995. Ecosocietal restoration reestablishing humanity’s relationship with natural systems.Environment: Science and Policy for Sustainable Development, 37(5), pp.4-33.
Choi, Y.D., 2004. Theories for ecological restoration in changing environment: toward ‘futuristic’restoration. Ecological research, 19, pp.75-81.
Choi, Y.D., Temperton, V.M., Allen, E.B., Grootjans, A.P., Halassy, M., Hobbs, R.J., Naeth, M.A. and Torok, K., 2008. Ecological restoration for future sustainability in a changing environment. Ecoscience, 15(1), pp.53-64.
Costanza, R., 2012. Ecosystem health and ecological engineering. Ecological Engineering, 45, pp.24- 29.
Costanza, R. and Mageau, M., 1999. What is a healthy ecosystem? Aquatic ecology, 33(1), pp.105-115. Davies, S.P. and Jackson, S.K., 2006. The biological condition gradient: A descriptive model for interpreting change in aquatic ecosystems. Ecological Applications, 16(4), pp.1251-1266.
Ellis, E.C., 2011. Anthropogenic transformation of the terrestrial biosphere. Philosophical Transactions of the Royal Society A: Mathematical, Physical and Engineering Sciences, 369(1938), pp.1010-1035.
Evers, C.R., Wardropper, C.B., Branoff, B., Granek, E.F., Hirsch, S.L., Link, T.E., Olivero-Lora, S. and Wilson, C., 2018. The ecosystem services and biodiversity of novel ecosystems: A literature review. Global ecology and conservation, 13, p.e00362.
Gobster, P.H., 2001. Visions of nature: Conflict and compatibility in urban park restoration. Landscape and urban planning, 56(1-2), pp.35-51.
Gobster, P.H. and Barro, S.C., 2000. Negotiating nature: Making restoration happen in an urban park context. Restoring Nature: Perspectives from the Social Sciences and Humanities, pp. 185-208. Island Press. Washington, DC.
Google Maps, 2021. Map of Montrose Point, Chicago, IL. Available: https://www.google.com/maps/place/Montrose+Point+Bird+Sanctuary/@41.964173,- 87.6398797,2697m/data=!3m1!1e3!4m5!3m4!1s0x880fd3e8cb519091:0x224ad894992736c2!8m2! 3d41.9631998!4d-87.6331366 (September 2021).
Higgs, E.S., 1997. What is Good Ecological Restoration? Conservation biology, 11(2), pp.338-348.
Hobbs, R.J., 2004. Restoration ecology: the challenge of social values and expectations. Frontiers in Ecology and the Environment, 2, pp.43-48.
Hobbs, R.J., Arico, S., Aronson, J., Baron, J.S., Bridgewater, P., Cramer, V.A., Epstein, P.R., Ewel, J.J., Klink, C.A., Lugo, A.E. and Norton, D., 2006. Novel ecosystems: Theoretical and management aspects of the new ecological world order. Global ecology and biogeography, 15(1), pp.1-7.
Jackson, L.L., Lopoukhine, N. and Hillyard, D., 1995. Ecological restoration: a definition and comments. Restoration Ecology, 3(2), pp.71-75.
Kareiva, P., Watts, S., McDonald, R. and Boucher, T., 2007. Domesticated nature: Shaping landscapes and ecosystems for human welfare. Science, 316(5833), pp.1866-1869.
Lackey, R.T., 2001. Values, policy, and ecosystem health: Options for resolving the many ecological policy issues we face depend on the concept of ecosystem health, but ecosystem health is based on controversial, value-based assumptions that masquerade as science. BioScience, 51(6), pp.437-443.
Martin, P.S., 1973. The Discovery of America: The first Americans may have swept the Western Hemisphere and decimated its fauna within 1000 years. Science, 179(4077), pp.969-974.
Mitsch, W.J., 1996. Ecological engineering: A new paradigm for engineers and ecologists. Pages 114- 132 in Schulze, P.C. (Ed.), Engineering within Ecological Constraints. National Academy Press, Washington, DC, pp. 114–132.
Mitsch, W.J. and Jørgensen, S.E., 1989. Ecological engineering: An introduction to ecotechnology. U.S. Department of Energy, Office of Scientific and Technical Information. Washington, DC.
Mitsch, W.J. and Jørgensen, S.E., 2003. Ecological engineering: A field whose time has come. Ecological engineering, 20(5), pp.363-377.
Odum, E.P., 1985. Trends expected in stressed ecosystems. Bioscience, 35(7), pp.419-422. Odum, H.T. and Odum, B., 2003. Concepts and methods of ecological engineering. Ecological Engineering, 20(5), pp.339-361.
Page, J., 2016. Tidy or Tangled: How People Perceive Landscapes. Partnership for Action Learning in Sustainability (PALS). University of Maryland, Baltimore.
Palmer, M., Bernhardt, E., Chornesky, E., Collins, S., Dobson, A., Duke, C., Gold, B., Jacobson, R., Kingsland, S., Kranz, R. and Mappin, M., 2004. Ecology for a crowded planet.
Pratt, J.R., 1990. Aquatic community response to stress: Prediction and detection of adverse effects. In Aquatic toxicology and risk assessment: Thirteenth volume. ASTM International.
Rapport, D.J., Regier, H.A. and Hutchinson, T.C., 1985. Ecosystem behavior under stress. The American Naturalist, 125(5), pp.617-640.
Rapport, D.J., Costanza, R. and McMichael, A.J., 1998a. Assessing ecosystem health. Trends in ecology & evolution, 13(10), pp.397-402.
Rapport, D.J., Gaudet, C., Karr, J.R., Baron, J.S., Bohlen, C., Jackson, W., Jones, B., Naiman, R.J., Norton, B. and Pollock, M.M., 1998b. Evaluating landscape health: integrating societal goals and biophysical process. Journal of environmental management, 53(1), pp.1-15.
Rapport, D.J. and Singh, A., 2006. An ecohealth-based framework for state of environment reporting. Ecological Indicators, 6(2), pp.409-428.
Schaeffer, D.J., Herricks, E.E. and Kerster, H.W., 1988. Ecosystem health: I. Measuring ecosystem health. Environmental Management, 12(4), pp.445-455.
Schindler, D.W., Mills, K.H., Malley, D.F., Findlay, D.L., Shearer, J.A., Davies, I.J., Turner, M.A., Linsey, G.A. and Cruikshank, D.R., 1985. Long-term ecosystem stress: The effects of years of experimental acidification on a small lake. Science, 228(4706), pp.1395-1401.
Schindler, D.W., 1987. Detecting ecosystem responses to anthropogenic stress. Canadian Journal of Fisheries and Aquatic Sciences, 44(S1), pp.s6-s25.
Schweitz, L.F., 2017. Reforming Our Visions of City Nature. Intersections, 2017(46), p.7.
United States Department of Energy, 2021. Ecological Engineering Remedies Presentation Given at International Atomic Energy Agency Conference. Available: https://www.energy.gov/lm/articles/ecological-engineering-remedies-presentation-given-international- atomic-energy-agency (September 2021).
Vitousek, P.M., Mooney, H.A., Lubchenco, J. and Melillo, J.M., 1997. Human domination of Earth’s ecosystems. Science, 277(5325), pp.494-499.
Westphal, L.M., Gobster, P.H., and Gross, M., 2010. Page 208-217 in M. Hall (editor). Restoration and History: The Search for a Usable Environmental Past. Routledge, New York, NY.