1 Science and Practice
The first chapter in the fundamental techniques group is on science and practice. Science and practice are unique and yet linked together in the goal of improving ecological conservation. This chapter on science and practice sets the stage for reviewing the conservation techniques covered in the remaining chapters of this book. We will define the meanings of science and practice, explore parallels, differences, and challenges, and end with a case study exploring the roles of science and practice in a long legal battle over whether to build a pump storage plant in the face of Storm King Mountain on the Hudson River.
SCIENCE AND PRACTICE DEFINED
Science has a tradition of showing and promoting progress. Science is the use of observation, study, and experimentation to derive knowledge about the nature or principles of what is being studied. Ecological science is aimed at understanding nature, and forming principles that explain the processes and properties of environmental systems. The basic scientific method includes (Figure 1.1):
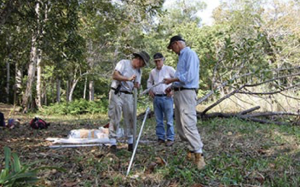
1) Reporting an observation or finding; 2) Developing a hypothesis to explain the observation or finding; 3) Testing the hypothesis using data and analyzing the results to determine if the data are significantly different than expected values under the null hypothesis; 4) Interpreting the results and comparing those with the results of other studies; and 5) Forming conclusions in the context of theory or principles. This scientific method shapes research and scientific activity, and also serves as the basis for making progress. Science contributes to ecological conservation by generating facts, improving understanding, and providing the ability to explain natural phenomena.
Conservation practices are aimed at protecting and managing the environment (Figure 1.2). Conservation practitioners must assess the consequences of their decisions through objective analysis of current conditions, plans for possible actions, and subjective evaluation of the significance of any anticipated changes. Practitioners must anticipate changes in order to formulate a best estimate of the expected outcome, without having full knowledge of, or confidence in, what will actually happen. The basic management method identifies goals and objectives, establishes baseline conditions, reviews options for action, anticipates changes under each option by collecting, analyzing and interpreting data to determine if these changes will be significant, documents expected benefits, and chooses a plan for action. Though this sequence is often performed with uncertainty and knowledge gaps, it is necessary for deciding what to do. Conservation practice contributes to the resolution of environmental problems by decisions, planning improvements, and anticipating future outcomes.
DIFFERING PERSPECTIVES OF SCIENCE AND PRACTICE
There are ways that science and practice fundamentally differ. Science is traditionally reductionist in that it aims to deal with hypotheses and specific questions. Scientists try to eliminate other factors that can confound responses to treatments. Recent trends in science, however, emphasize interdisciplinary collaboration and a more system-wide orientation. Practitioners are generally holistic in their approach, and consider the big picture so that all perspectives and facts are taken into account. Practitioners often work at a broad scale seeking to assemble, analyze and evaluate all the information associated with actions or change. Thus, science and practice may be converging with current trends, but traditionally diverge in scope.
One polarizing notion is the significance of an outcome. Significance in science is quantitatively estimated as the probability that an observation deviates from a hypothesis, but science tolerates only very small probabilities. More specifically, science uses the probability level associated with rejecting a true null hypothesis. This is described as the probability of having a type I error (P ≤ 0.05). These data that form a type I error deviate from predictions under the null hypothesis, enough to be considered consistent with the alternative hypothesis. Significance in practice is more subjective and is often based on agency policies, public concerns, legal standards and responsibilities, personal preferences, and past case histories. Significance is not commonly estimated in practice. Instead, over time, a judgment is made as to whether practices are working.
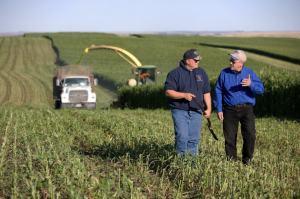
Finally, achievement and progress are viewed differently in science and practice. In science the published scientific literature shows progress through changing principles and advancements in understanding. Major results are frequently challenged, tested and revised, and the theories, principles, and paradigms developed by the synthesis of these findings provide a progression of ideas and accepted truths which accumulate in the scientific literature. These widely accepted principles of science are presented in books and taught in schools. That is why scientists are expected to publish articles and present lectures. For practitioners, the basis for decisions are often poorly documented in a systematic manner, and few true management journals, books and courses exist. Little time is afforded to communicate methods or results of management studies. Thus, study results and predictions are rarely checked or verified. Rather, institutional experience is derived from successes, and people with institutional experience pass on those lessons inside an organization. Laws, policies, and practices often change for reasons other than management success or failure.
SHARED PERSPECTIVES OF SCIENCE AND PRACTICE
Scientists and practitioners have much common ground as well. Many have similar reasons for directing their careers to include the environment early in their lives. They are often science-oriented, with a technical education focused on a single discipline. They make use of studies to accomplish their work, with a heavy reliance on data and objective analyses. Many have a high regard for precise, quantitative results underpinned with biological knowledge. They often find that written material is the primary measure of productivity and that those writings are almost always subjected to independent reviews. And, most eventually encounter frustrations due to restrictions on topics and priorities often because of funding availability.
CHALLENGES WITH MERGING SCIENCE AND PRACTICE
Ecological conservation is informed by science. Science provides the facts, guidance, and methods to protect and improve our environment. Conservation is the act of putting science into practice. However, science and practice are not necessarily close in perspective, values, methods, and considerations. The relationship between science and practice varies by approach, though a close coordination between science and practice is seen as a key to success in ecological conservation. The differences in thinking between science and practice seem to be a product of the workplace, on-the-job demands, and the local culture (Pouyat 1999). Acknowledging the differences between science and practice across conservation techniques can provide a broader and potentially more effective approach to ecological conservation.
Both scientists and practitioners want to make a better world and solve environmental problems. Both see the need for a merger of science and practice to develop effective decisions, plans, policies, and make progress on ecological challenges. Science offers facts, analytic methods, and understanding of complex ecological systems.
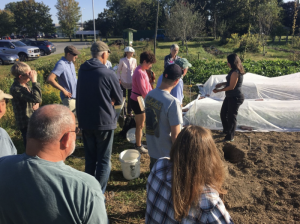
Practitioners have the capacity for taking action, identifying feasible options, and making implementation work. Ultimately, ecological conservation should not be treated as a singular perspective. Instead, we should foster the recognition of different strengths and options for making progress by exploring science and practice within many different conservation techniques. Merging science and practice for each ecological conservation technique is challenging because of the inherent differences across applications (Figure 1.3). However, there are expected benefits from integrating science and practice, and many are promoting this as the way forward to improve our environment. In theory, combining these two distinct pursuits of science and practice will promote improvements in ecological conservation, and over time trends will emerge regarding the best way to conserve the environment.
WHAT EACH SIDE NEEDS FROM THE OTHER
Generally, what practitioners need from scientists is a simple, widely applicable technique that will detect the health of the environment. Ideally, this technique would involve steps that are clear and fully described so they can be followed by people with little or no training (Cairns 1985). If a device is involved, it should be inexpensive, readily available, and portable (Cairns 1985). The technique should provide results that can be obtained immediately and are easily understood (Cairns 1985). Results from methods and models should also be reliable, credible, provide guidance on meaning, and take into account key factors, processes, mechanisms, and structural properties that represent the essential characteristics and functions of systems and species. Practitioners often need this information to produce general “rules-of-thumb” and track important variable levels or system conditions that define limits of health (e.g., thresholds or tipping points).
Generally, what scientists need from practitioners is guidance regarding the balance between research that is intensive and extensive. Intensive research experimentally investigates one or a few factors and extrapolates findings to a broader context. This is the process of induction, in which we use specific findings to make general conclusions (e.g., Keeling Curve). Extensive research is broad-based and looks for patterns that suggest processes of importance. This is the process of deduction, in which we use general principles to explain specific observations. Scientists also need guidance regarding the divergence between research aimed at understanding the complexity of nature and research aimed at developing indicators or simple measures of environmental health. Additionally, scientists need guidance on appropriate scale of investigation which can be thought of as the geographic scope of interest (e.g., microhabitat to landscape ecology). Finally, scientists need more guidance on what will be important 5-10 years from now, as well as crises that exist today.
EXAMPLES OF SCIENCE IN USE BY CONSERVATION ORGANIZATIONS
Many examples exist of science successfully integrated into practice at conservation organizations. For example, the Nature Conservancy (2021a; 2021b) says on their website: “Science matters, especially at this critical turning point for nature. Our work is grounded in science.” The mission of The Nature Conservancy is to “preserve the plants, animals, and natural communities that represent the diversity of life on earth by protecting the lands and waters they need to survive,” and they have currently preserved land on almost all continents around the world.
Another example is Conservation International (2021a; 2021b) whose website says: “Science has always guided our work, and we rely on science and evidence as the foundation of conservation.” The mission of Conservation International is “building upon a strong foundation of science, partnership and field demonstration, Conservation International empowers societies to responsibly and sustainably care for nature, our global biodiversity, for the well-being of humanity.” These are just two of many such organizations that use science as the basis for their conservation actions.
EXAMPLES OF SCIENCE IN USE BY GOVERNMENTAL ORGANIZATIONS
There are also many examples of science successfully integrated into practice within governmental organizations (Figure 1.4). For example, the former director of the United States Fish and Wildlife Service (USFWS), H. Dale Hall, was quoted (2008) to have said, “Science underpins everything we do as an agency.”
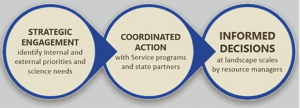
The National Oceanic and Atmospheric Organization (NOAA) put science directly in its mission statement by proclaiming science as one of its three goals: Science, service and stewardship (2021).
These are just two of many such governmental organizations that use science as the basis for their decision-making and actions.
CASE STUDY: PEACE TREATY ON THE HUDSON RIVER
In 1963, the Consolidated Edison Company (Con Ed) proposed to embed the world’s largest pump storage plant into the face of Storm King Mountain (Figure 1.5) on the Hudson River (Marist College Cannavino Library 2021).
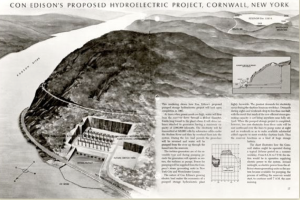
Pumped storage hydropower (PSH) is a type of hydroelectric energy storage system configured with two water reservoirs at different elevations, that can generate power as water moves down from one reservoir to the other (discharge), passing through a turbine (United States Department of Energy 2021). PSH acts similarly to a giant battery because it can store power and then release it when needed (United States Department of Energy 2021). This proposal by Con Ed generated the longest, most expensive, most litigated, and most important environmental controversy in United States history up to that point (Barnthouse et al. 1984). It also spurred the start of the United States environmental movement with the development of many environmental organizations, such as Scenic Hudson (Marist College Cannavino Library 2021), Riverkeeper (2002), Clearwater, Natural Resources Defense Council, the Hudson River Foundation, and the environmental consulting profession (Lubchenco 1998).
Opposition to Con Ed’s plan was mounted by several groups. People who valued the aesthetics of the Hudson River, such as the Hudson River School artists (Ferber 2009), formed one group (Figure 1.6). Those opposed to the potential environmental effects formed another group. Those concerned about the impact to striped bass populations formed a third group.
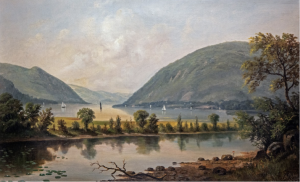
There were already a number of existing power generating facilities on the Hudson River (Figure 1.7).
Science played an important role in the decision-making process about whether to let Con Ed’s plan move forward. The core issue was the effect of electric generation on striped bass populations. There was no doubt that electric generation plants killed striped bass through impingement and entrainment. Impingement is the physical contact of a fish with a barrier structure (e.g., screen) due to intake velocities which are too high to allow the fish to escape (West Virginia Department of Environmental Protection 2021). Entrainment is the unwanted passage of fish through a water intake, generally caused by an absent or inadequate screen surrounding the water intake (West Virginia Department of Environmental Protection 2021). The question under investigation was whether losses through impingement or entrainment would have a significant impact on the striped bass population. Scientists used mathematical population models as environmental assessment tools.
Two models were developed. The first model was developed by a consulting company hired by Con Ed. It compared average daily water use versus daily tidal flow and assumed that fish were uniformly distributed in the water. The results of the model indicated there would likely be negligible impact of the pump storage plant on fish populations. The second model was developed by Oak Ridge National Laboratory. It predicted that small fish would accumulate at the salt front and circulate on an “endless belt” near the power plant. The results of this model predicted that 30-50% of the population would be killed (Barnthouse et al. 1984).
Both models were attacked as being oversimplified and unrealistic (Barnthouse et al. 1984). It was argued by a witness testifying on behalf of Con Ed that, if tidal circulation and cooling water withdrawal were modeled more realistically, impact estimates would be reduced by 75%. So, a more elaborate hydrodynamic model was developed with non-uniform spatial distribution of organisms and ‘migration factors’ (used to model the movement of juveniles). These migration factors became the next focus of debate.
In its initial licensing decision, the Atomic Safety and Licensing Board (ASLB) decided that the Indian Point plant was the cause of a substantial reduction in the striped bass population, and ordered that cooling towers be built. This decision was appealed by Con Ed, who won using the hydrodynamic model.
New models were developed by both sides Models succeeded models but the results stayed the same. The models developed by the utility companies showed negligible impact, while the models developed by regulatory agencies predicted serious fish population depletion. Neither side could be defended conclusively.
There were two main failings of the models. The first was that hydrodynamics did not actually predict the distribution of fish.
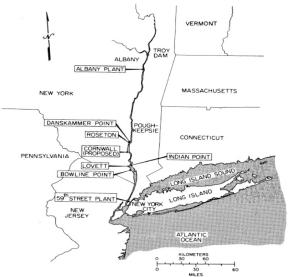
The models were forced to match known distributions, but then did not simulate hydrodynamics properly. The second was that population compensation, the idea of allowing entrainment mortality to be offset by a decrease in the natural mortality rate of the non-entrained striped bass, was not well understood. Without accurate estimates of compensation, any of the models could be forced to predict either a large or a small impact with virtually equal plausibility (Swartzman et al. 1977).
Since the models were not proving helpful, direct impact assessment was tried next. Empirical transport accounting utilized extensive field data and estimated losses by river regions. Estimated reductions in the size of the 1974 and 1975 year classes ranged from 12% to 14% according to utility scientists, and from 12% to 22% according to regulatory agency scientists (Barnthouse et al. 1984). The data also showed that, for most fish, impingement was less of an issue than entrainment. A focus on reductions in abundance of individual year classes eliminated the need to make and defend long-term impact predictions. It also eliminated any remaining hope of resolving the issue of whether cooling towers were needed to ensure the long-term viability of Hudson River fish populations (Barnthouse et al., 1984). Thus, after tens of millions of dollars and a decade of study, it was still not possible to know the effects of the proposed pump storage plant on striped bass populations with confidence. In the end, as part of the “Hudson River Settlement Agreement” in 1980, the utility companies agreed to implement flow reductions and scheduled shutdowns as an alternative to cooling towers to reduce entrainment of fish species. They also funded ongoing environmental monitoring, the creation of the Hudson River Foundation, and agreed to operate a striped bass hatchery on the river. And, the idea of a pump storage plant at Storm King Mountain was abandoned. In return, the environmental organizations and United States Environmental Protection Agency (USEPA) dropped the requirement of closed system cooling, which would have necessitated the building of six costly cooling towers. Additionally, the USEPA would allow the plants to continue operating as they have in the past. This agreement stands as a model for balancing economic and environmental needs.
Lessons learned: 1) Conclusive results were not possible even with a simple case looking at one species (Striped bass) in one river (Hudson River) with known loss mechanisms; 2) In this case, simple empirical analyses were more useful than simulation models; 3) Decisions need to be made despite uncertainties; 4) The ultimate question, “what will be the long-term effects of once-through cooling on Hudson River fish populations?” was unanswerable; 5) An alternative question, “what are the available methods of reducing the impact of once-through cooling, and how can they be most effectively deployed?” enabled scientists to make a positive contribution.
SUMMARY
Science and practice are unique and yet work together toward the goal of improving ecosystem health through environmental conservation. Science provides the knowledge used to create decisions that lead to action. Practice is the act of managing and conserving the environment. These two fields often have different perspectives. When they work together successfully, their collaboration can prove effective at improving ecosystem quality.
REFERENCES
Barnthouse, L.W., Boreman, J., Christensen, S.W., Goodyear, C.P., Van Winkle, W. and Vaughan, D.S., 1984. Population biology in the courtroom: The Hudson River controversy. BioScience, 34(1), pp.14- 19.
Cairns, J. Jr., 1985. Just give me a freeze dried, talking fish on a stick. Journal of the Water Pollution Control Federation 57:980.
Conservation International, 2021a. About Conservation International. Available: https://www.conservation.org/about (September 2021).
Conservation International, 2021b. Conservation International homepage. Available: https://www.conservation.org/ (May 2021).
Ferber, L.S., 2009. The Hudson River School: Nature and the American Vision. Skira.
Lubchenco, J., 1998. Entering the century of the environment: A new social contract for science. Science, 279(5350), pp.491-497.
Marist College Cannavino Library, 2021. The Marist Environmental History Project: Dedicated to Pre- serving Materials Concerning the Scenic Hudson Decision. Available: http://cannavinofaculty.blogspot.com/2012/03/marist-environmental-history-project.html (September 2021).
National Oceanic and Atmospheric Organization, 2021. Our mission and vision. Available: https:// www.noaa.gov/our-mission-and-vision (September 2021).
National Science Foundation, 2021. Ancient Maya Practiced Forest Conservation. Available: https:// www.nsf.gov/news/mmg/mmg_disp.jsp?med_id=73037&from=mmg (October 2021).
New York State Office of General Services, 2021. Mid-Hudson works on view. Available: https://empirestateplaza.ny.gov/hall-new-york/mid-hudson (September 2021).
Pouyat, R.V., 1999. Science and environmental policy—making them compatible. BioScience, 49(4), pp.281-286.
Riverkeeper, 2002. Hudson River Settlement Agreement (HRSA). Available: http://www.riverkeeper.org (September 2021).
Swartzman, G., Deriso, R. and Cowan, C., 1977. Comparison of simulation models used in assessing the effects of power-plant-induced mortality on fish populations. In Proceedings of the Conference on Assessing the Effects of Power-Plant-Induced Mortality on Fish Populations (pp. 333-361). Pergamon.
The Nature Conservancy, 2021a. Articles of Incorporation. Available: https://www.nature.org/en-us/about-us/who-we-are/accountability/articles-of-incorporation/ (September 2021).
The Nature Conservancy, 2021b. Our Science. Available: https://www.nature.org/en-us/about-us/who- we-are/our-science/?vu=r.v_tncscience (September 2021).
United States Department of Agriculture, 2021. Conservation planning. Available: https://www.nrcs.usda.gov/wps/portal/nrcs/detail/nd/technical/cp/?cid=nrcseprd895612 (October 2021).
United States Department of Energy, 2021. Pumped storage hydropower. Available: https://www.energy.gov/eere/water/pumped-storage-hydropower (September 2021).
United States Fish and Wildlife Service, 2021. Conserving the Nature of America. Available: https://www.fws.gov/news/ShowNews.cfm?_ID=3372 (September 2021).
Warren County Soil and Water Conservation District, 2021. Community conservation program. Available: https://warrenswcd.org/ (October 2021).
West Virginia Department of Environmental Protection, 2021. Best Management Practices for Entrainment and Impingement Prevention. Available: https://dep.wv.gov/oil-and-gas/Water %20Management/Documents/Entrainment%20and%20Impingement%20Prevention %20BMPs_Final.pdf (September 2021).