4 Rewilding
The first chapter in the biologically-focused techniques group centers on the idea of rewilding. Rewilding has at its core the goal of reestablishing species and interactions in an effort to restore natural ecosystems. This chapter will cover some background on rewilding, the theoretical basis for its use, examples of implementation, and will end with a case study on gray wolf (Canis lupus) reintroductions in Yellowstone National Park.
BACKGROUND ON REWILDING
The concept of rewilding is grounded in the notion that to have truly natural ecosystems, ecological processes must be reestablished, allowing nature to create or rebuild these ecosystems. Dynamic interactions among species are regarded as the fundamental bases of natural processes that support natural ecosystems. Large carnivores and herbivores are seen as necessary to shape the flora and physical characteristics of natural environments (Asner et al. 2009). Many large species of carnivores and herbivores were extirpated by humans beginning 150,000 years ago, resulting in altered ecosystem functions and attributes (Martin 2005). Estimating an ecosystem’s original functions and processes becomes a near impossible task due to the impact of thousands of years of human activity. Intensive management of parks and landscapes are unlikely to yield a fully natural setting as ecosystems have undergone extensive, long-term alterations (Sutherland 2002).
Rewilding sets out to recreate a full assemblage of interacting species within a specific ecosystem. In essence, the rewilding concept aims to assemble the “biological pieces” and then let nature rebuild populations to reestablish the interrelationships which support and maintain the assemblage and its ecosystem. Top predators constrain herbivore diversity and abundance. The composition of herbivores shape plant cover and the physical attributes of their habitats. Competition among both flora and fauna also determines community composition and its effect on the broader landscape. A core principle is that biotic processes will result in an ecosystem that is more natural than we can predict and create ourselves. Consequently, the rewilding concept may seem unrealistic and risky to implement. However, rewilding efforts have been implemented and some have demonstrated very profound results in reestablishing biotic and abiotic ecosystem characteristics. Some of the principles of ecology justify this approach and some applications of this concept have demonstrated success for conservation and restoration purposes.
A basic and distinctive attribute of the rewilding concept is reestablishing large predators and herbivores that were targeted by early people. Restoring large species has primarily been done by relocating species to a new setting where a full community is to be built. We have several examples of successfully rebuilt natural communities (Marris 2009; Ismail 2011; Taylor et al. 2019; Pettersson and de Carvalho 2021; Segar et al. 2021) including: wolves (Canis lupus) brought back to Yellowstone National Park (USA); houbara bustards (Chlamydotis undulata), sand gazelle (Gazella subgutturosa marica) and Arabian oryx (Oryx leucoryx) reintroduced to Mahazat as-Sayd reserve (Saudi Arabia); giant anteaters (Myrmecophaga tridactyla), tapir (Tapirus terrestris), collared peccaries (Pecari tajacu), and pampas deer (Ozotoceros bezoarticus) reintroduced to the Iberian wetlands (Argentina); and little-spotted kiwi (Apteryx owenii) and tuatara (Sphenodon punctatus) released in Zealandia (New Zealand). However, many large predators and herbivores are extinct and were eradicated by humans tens of thousands of years ago. Where an exact species match is not possible, rewilding promotes the use of analog species to complete a full community and reestablish ecological processes. Introducing non-native species that have strong effects on biota, and potentially the physical environment, may seem contrary to conservation practices. However, it is consistent with the core idea of rewilding that a natural environment is the product of ecological processes. This approach has been implemented with Heck cattle (Bos taurus primigenius) and Konik horses (Equus ferus caballus) to replace extinct aurochs (Bos taurus) and tarpans (Equus ferus) in Oostvaardersplassen reserve (Netherlands; Vera 2009), tundra musk oxen (Ovibos moschatus) have been used to replace the extinct Siberian musk oxen (Ovibos palantis) in the Siberian steppe (Russia; Parker et al. 2010), and Aldabran giant tortoises (Aldabrachelys gigantea) have replaced the extinct giant Cylindraspis tortoises (Cylindraspis sp.) in the Mascarene Islands (Mauritius; Griffiths et al. 2010).
One privately owned estate (owned by English multimillionaire conservationist Paul Lister), called Alladale Wilderness Reserve in the Scottish Highlands, is being managed to restore it to its natural state by increasing the tree cover of the valley, reintroducing native animals which are no longer found in Great Britain, restoring damaged peatlands, encouraging biodiversity, and promoting education through ecotourism (Alladale Wilderness Reserve 2021). Alladale Wilderness Reserve is currently the United Kingdom’s largest wilderness rewilding area. It hosts horses (Equus sp.) as a proxy for tarpan (Equus ferus), bovine (Tragelaphus sp.) as a proxy for aurochs (Bos taurus), European bison (Bison bonasus), European elk (Alces alces alces), wild cats (Felis silvestris), red squirrels (Sciurus vulgaris), and has plans to reintroduce wild boar (Sus scrofa), bear (Ursus sp.), and lynx (Lynx sp.) (Sandom et al. 2019).
Starting in 2005, Donlan et al. (2005; 2006) focused worldwide attention on the rewilding approach by proposing a large reserve in the central United States with African lions (Panthera leo), African elephants (Loxodonta africana), and other non-native species as proxies for long lost native species. The purpose of these species’ introductions was to re-create the extinct megafauna assemblages that were eradicated by the first Americans 13,000 years ago. They termed this strategy Pleistocene rewilding, and made the case that it is the only way to restore a fully natural grassland landscape (Figure 4.1). Pleistocene history and taxon substitutions can provide benchmarks for restoration not only by the presence or absence of species but by the presence or absence of species interactions, which constitutes the true functional fabric of nature. The time period of 13,000 years ago corresponds roughly with the arrival of the first Americans from Eurasia and constitutes a less arbitrary baseline than the PreColumbian/European standard often used. For 200 million years, large carnivores and megaherbivores were dominant features of most ecosystems. With a few exceptions, primarily in Africa, these animals became functionally extinct worldwide by the late Pleistocene. Vegetation communities have shifted and changed before and after the late Pleistocene, but the major missing component in contemporary ecosystems is large vertebrate herbivores. The Great Plains of the United States was suggested as a prime location since human populations in the region are declining and this area could support a vast, privately-owned and managed “ecological history park.” The park would provide animals currently in captivity with an alternative: large natural settings, vast protected areas to roam, and live vegetation/ prey. It was a bold proposal for conservation, one more optimistic than many of the proposals put forth by modern day environmentalists, and it started a debate about the rewilding concept (Rubenstein et al. 2006).
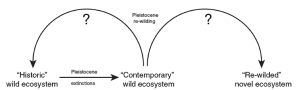
Another approach that has received considerable attention is de-extinction (Novak 2018). De-extinction is an amalgum of genetic engineering, stem cell research and conservation. Essentially, de-extinction is the restoration or revival of a species by manipulation of their genetic material from an artifact source. The rationale and support for de-extinction parallel the same premises as Pleistocene rewilding: an attempt to restore ecosystems and habitats to a more natural prior state, increase biodiversity, and from a moral and ethical standpoint, undo the damage done by humans that resulted in the extinction of the species. De-extinction reinvigorates the same issues as rewilding in regard to the unintended and detrimental effects that may result from the introduction of a species that is no longer part of the current ecosystem.
A related issue and concern is: what is natural? Considering the variability and evolutionary changes that define nature, what lapse in time between between the absence of a species and reintroduction to an ecosystem is realistic? Opponents to de-extinction cite potential issues with reintroduced species exerting undue pressure on the environment and other species through competition for resources. Further, reintroduced species may serve as vectors for disease and parasites. Advocates of de-extinction support a continued focus on identifying potential studies and mitigation protocols to minimize and eliminate these risks. To date, proponents of de-extinction claim the successful creation of a frog embryo (Rheobatrachus silus) that has been extinct for 30 years and the genetic material of Pyrenean Ibex Capra (pyrenaica pyrenaica) has also been resurrected for a few minutes (Banks and Hochuli 2017). Similar attempts are underway to apply genetic technology to resurrect some of the most iconic extinct species that have been lost in recent times, including passenger pigeons (Ectopistes migratorius), thylacines (Thylacinus cynocephalus) and mammoths (Mammuthus sp.; Banks and Hochuli 2017). The discourse and debate on the pros, cons and feasibility of de-extinction continue.
THEORETICAL CONCEPTS BEHIND REWILDING
There is a set of principles of ecological science that support the rewilding approach. Top-down effects of predators on herbivores and beyond can have a strong influence all the way down to the vegetation cover within a landscape. The interactions of species in food chains and food webs can shape the biota, and different biota can shape a variety of ecosystem features including physical properties. Highly influential species can alter entire communities and have widespread effects on the nature of ecosystems Estes et al. 1998). Finally, the sequential effects across trophic levels can shape the biota and cascade down to the levels that alter the abiotic properties of an ecosystem (Hansen and Galetti 2009). Most rewilding efforts are predicated on the top-down trophic cascade effects on ecosystems that large carnivores and herbivores can trigger. These ecological principles complement ideas of rewilding, and set the stage for considering how to promote a natural restoration agenda.
The Hairston-Smith-Slobodkin (HSS) hypothesis (Hairston et al. 1960) posits that carnivores are responsible for a green world because predation limits the abundance of herbivores. The basis of this thesis is that any population not limited by its food supply must be limited by predators. Herbivores are commonly reduced by predation below density levels that would be limited by an adequate supply of plants. And, it is common that in today’s suburban and urban landscapes, herbivores like whitetailed deer (Odocoileus virginianus) lack predators. Frequently, this species becomes over abundant in these areas leading to winter starvation and heavily browsed vegetation in developed settings. Thus, by consuming herbivores, predators actually benefit plant life by reducing browsing. This hypothesis has been tested repeatedly and often supported (Ripple and Beschta 2012), and has now become one of the principles of ecological science. This principle also directly supports the rewilding approach by justifying why a full complement of biotic community members is necessary to support a fully functioning ecosystem shaped by natural processes.
A meta-analysis (Schmitz et al. 2000) of 60 tests of the HSS hypothesis in terrestrial settings showed that carnivores alter plant cover indirectly through predation on herbivores. Analyses of a majority of these studies showed that plants were changed by the removal of carnivores. Though these studies included a variety of vertebrate and invertebrate carnivores, the type of carnivore did not diminish the top-down effect on plants. The conclusion, beyond supporting the HSS hypothesis, indicates that top predators have pervasive effects across an ecosystem. A clear case of this idea was shown on newly created islands in a large Venezuelan reservoir (Terborgh et al. 2001). When the reservoir was filled with water, it trapped partial communities on the islands. On predator-free islands, herbivores became hyper-abundant and not only depressed plant abundance but shifted the plant cover to taxa that were resistant to grazing. These results indicate that partial communities, especially those lacking predators, can result in biologically impoverished plant cover. Subsequently, these islands looked quite different of significant changes in vegetation cover. Additionally, top predators have been shown to be important for maintaining the diversity of smaller predators. In the absence of top predators, lower trophic level carnivores proliferate and imperil their prey (Johnson et al. 2007).Thus, the bottom line is: without the presence of a full complement of species, we will not have a natural community in ecosystems, parks, or protected areas.
The concept of the predators-herbivores-plants interrelationship represents a simple food chain. More complex is the notion of a food web, first introduced by Elton (1927) (Figure 4.2). Food webs show connections among many species through predation and competition for the same food resource (Paine 1980). These species and connections can be organized by trophic levels and linkage strengths (Paine and Schindler 2002). Species with strong interactions can significantly shape community, and their absence can result in an altered community with very different properties. Moreover, changes in communities at specific locations can alter the properties of an entire ecosystem. These strongly influential species are often labeled keystone species (Paine 1969). Links within trophic levels commonly designate competition, which can also shape community composition through time. The concepts of a food web with species interactions and keystone species point to the need to have complex communities to attain a natural environment.
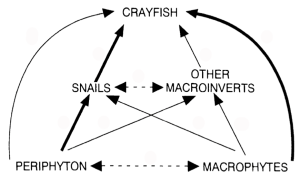
Food chains, food webs, and keystone species represent the pervasive effects various species can have across trophic levels in a cascading manner (Carpenter et al. 1985). The term cascade is appropriate here because these effects alternate across adjacent trophic levels, and this principle is termed a trophic cascade (Figure 4.3). The effects of trophic cascades can be strong and can influence the biological organization of an ecosystem and may extend to physical and chemical changes as well. For example, introducing predatory fish in lakes has the effect of reducing planktivore abundance, increasing zooplankton, decreasing phytoplankton, and increasing the transparency of the water. This trophic cascade results in differences in macrophyte abundance, nutrient cycling, and more (Schmitz et al. 2010). Shapiro and Wright (1984) were among the first to recognize the potential of altering food webs as a management tool and termed the approach biomanipulation. Biomanipulations are intentional modifications of trophic organizations that can force trophic cascades to shape the characteristics of an ecosystem (Shapiro and Wright 1984). This lake management approach was not connected to the idea of rewilding, but again it is based on the same ecological principles and processes. Similar trophic cascades have been documented for marine ecosystems (whales–otter–urchins–kelp), small freshwater bodies (mosquitoes–protozoa–bacteria), tropical forests (beetles– ants–insects–plants) and others (Pace et al. 1999). Cascading effects across trophic levels again indicate that top predators are needed to support conservation actions that seek to restore the natural properties of ecosystems.
Further effects of altered community composition have been reported, such as proliferation of small species, chemical cycling, vegetation structure, soil properties, and others. Predator effects, food chains and webs, keystone species, and trophic cascades all support the rewilding approach to achieve natural ecosystems through species interactions within a community and its environment. Early in human history our species hunted and eliminated many large predators and herbivores. Based upon the ecological principles just discussed, we can assume these actions prominently altered most landscapes. A consequence is that we may not recognize a natural ecosystem because changes occurred a very long time ago. The rewilding approach is one strategy that attempts to recover natural ecosystems by introducing species that can reestablish the necessary relationships endemic to an ecosystem’s community and habitat.
IMPLEMENTING REWILDING
The conservation movement has traditionally been dominated by defensive approaches such as saving wild spaces and adding restrictions to human activities. However, biodiversity continues to decline worldwide, so more comprehensive action,and programs may be needed beyond protecting places we deem as natural. Rewilding can be considered a proactive conservation strategy that attempts to restore natural environments by reinvigoration. It is an alternative to protection because it has a focus on re- creating natural environments by putting the ecological pieces together and letting nature take over. Additionally, megafauna often hold a particular fascination for the public who recognize that most large predators and herbivores were lost long ago. Rewilding can be an affirmative move in the public arena and can result in shifting some locations to more natural environments.
Top-down effects of predators, species interactions, and trophic cascades have been documented in a wide variety of habitats and may be critical to the formation of natural and stable ecosystems (Pace et al. 1999; Donlan et al. 2006; Donlan and Greene 2010). With the rise of human populations across the world, many large species became extinct and cannot be used to reestablish natural communities. The rewilding approach advocates replacing these extinct species with existing analog species to reinstitute natural processes that may restore ecosystems (Figure 4.4). An extreme version of this was proposed by Donlan et al. (2005; 2006) to recover natural grassland ecosystems on the Great Plains of North America. Their proposal includes importing lions, elephants, camels, Eurasian horses and other large vertebrates, as taxon substitutes for long lost native species that might reestablish lost evolutionary processes and ecosystem characteristics like natural plant cover. Reaction to this idea was both strongly positive and negative in the conservation and management communities. Supporters cited a proactive conservation agenda, intrigue surrounding the idea, and interest in large animals. Opponents focused on fear of the consequences, elitism and imperialism. Regardless of any particular agenda, it does introduce the idea of biological manipulations, including the use of non-native species to recreate natural features of a landscape that have since been lost.
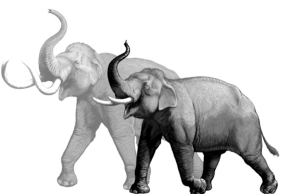
The rewilding strategy intends to go beyond natural properties of landscapes by including evolutionary effects on species. The effect of species interactions can limit abundances of small species, may deter invasive species, and shape the dispersion of species (Wallach et al. 2010). Species interactions like predation and competition can also shape the morphology and innate capabilities of species through natural selection. A clear example was raised by Donlan et al. (2005) for the pronghorn antelope (Antilocapra americana) which has incredible running speed that is no longer a fitness advantage since the demise of the American cheetah (Acinonyx trumani). Therefore the benefits of reintroducing species provide more than ecosystem and habitat recovery, they also influence the evolution of species characteristics, with ecotourism and extinction prevention of the American cheetah as additional benefits.
One distinctive feature of rewilding is the return of large predators to protected areas. While justified by the scientific evidence on predator effects, conservation interests often focus only on large preda- tors. The public is often inspired by predators because of their powerful and majestic appearance. Often predators serve as flagship species in conservation programs to garner public support. These species also push conservation toward thinking about large reserves because most top predators need a large range and an expansive habitat. Thus, large predators can serve to motivate and promote conservation efforts within the public arena, and expand the scope of conservation planning.
Research supports using top predators as an indicator of high biodiversity, environmental integrity, ecosystem productivity, and resilience; yet these predators are still dependent on species in lower trophic levels and are often the first to disappear when an ecosystem is disrupted. Larger predator species are impeded by habitat alterations and fragmentation, require prey that can be specialized, and suffer other vulnerabilities such as the accumulation of toxins. In a way this is the reverse of top-down effects because ecosystem degradation passes effects up through the trophic levels to the top predators. Therefore, top predators can serve as an umbrella species for conservation since they encapsulate conditions that support many other species, and they can serve as sentinel species for ecosystem disruptions from pollutants, habitat change, and fragmentation of the landscape (Sergio et al. 2008).
Rewilding is a controversial approach in the conservation movement because of the actions it entails, such as reestablishing keystone species, predators, and non-native species. This conservation strategy would require large tracts of land and significant public involvement. Rewilding can be risky since there is always the possibility of unanticipated consequences and catastrophic effects on native flora and fauna, their habitats, and even entire ecosystems. Failures such as these would be prominent in terms of public attention and could prove damaging to broader conservation interests. Restoring the long-lost past may be impossible in the modern world, and some feel that this direction is based more on sentiment than science. Finally, questioning the effectiveness of rewilding should be expected because we do not know against what conditions of the far past we can measure success. Also, rewilding can produce ecosystems that are more a product of its own implementation details than a reflection of the original or natural ecosystem it seeks to recreate. Doubts about this conservation technique continue to echo throughout conservation circles, particularly with respect to how anyone could determine whether rewilding successfully restored a natural ecosystem. Nevertheless, it is a conservation strategy that is currently used because of its potential benefits and also because of the rewilding efforts that have yielded positive results.
CASE STUDY: REINTRODUCING WOLVES INTO YELLOWSTONE NATIONAL PARK
The gray wolf (Canis lupus) is one of the most dominant predators in North America because this species hunts in packs and targets large herbivores. Wolves were intentionally eradicated in the Western United States as settlers brought in cattle, horses, and sheep which are natural prey for the wolf. Yellowstone National Park was formed in 1872 and from its origin prohibited domestic livestock grazing. Wolves maintained a small presence in the park area until the mid-1920s when they were extirpated from the Western United States. Wolves were reintroduced in the Yellowstone National Park area starting in 1995 in response to their endangered species status. The restoration of wolves in the Yellowstone National Park area completed the terrestrial community of large species, but was not considered to be rewilding. Since that conservation strategy was not identified in 1995. The reintroduction of wolves to this area was well studied and the findings indicate how pervasive the effects of a large predator can be in shaping an ecosystem (Figure 4.5) (Fortin 2005; Beschta and Ripple 2010). These findings were consistent with the scientific basis of rewilding. Thus, this case is used here as an example of rewilding, and shows how communities can change to reestablish a full ecosystem by bringing back its top predator.

Adjacent to the Yellowstone National Park, the upper Gallatin River valley is under the control of the United States Forest Service which prohibits livestock grazing to help maintain satisfactory forage conditions for the elk’s (Cervis elaphus) winter range. In 1919, when wolves were originally present in the area, the elk population was about 1,600. Then the wolf population was extirpated in the mid-1920s.

By the 1930s, elk numbers increased to around 2,500. Due to its increasing size, the elk population was reduced by culling, but this was opposed by the public. After the culling program was suspended, the herd size increased to 19,000 by 1968. At these high abundance levels, elk foraging conditions in their winter range became degraded due to high rates of herbivory. Starvation was common and the herd eventually shrank to about 1,000. Wolves were reintroduced in the park area in 1995. Many experts regard wolves as the most potent carnivore in North America. The effects of the reintroduced wolves were monitored, and many studies were conducted on all direct and indirect impacts of the wolves on the Gallatin River valley ecosystem.
The wolves initiated a trophic cascade by reducing elk numbers in the Gallatin River valley (Figure 4.6). Before wolves, elk freely grazed the riparian vegetation which was a favored food source. Once wolves returned to the valley, elk were subjected to a significant predation threat. The elk were vulnerable prey in the brushy and open terrain. Beyond reduced abundance, the elk changed their habitat use from the valley floor to the forested uplands. Behaviorally reduced use of the valley floor diminished riparian vegetation grazing. Slowly, after the mid-1990s, riparian vegetation such as aspen (Populus tremuloides) recovered along the Gallatin River (Figure 4.7) (Ripple and Beschta 2007).
The effects of wolf predation extended well beyond elk and riparian plants in many surprising ways. Changes to the river channel, groundwater levels, and soil conditions have been linked to reestablishment of wolves in the area. Prior to the reintroduction of wolves, heavy grazing of riparian vegetation by elk reduced the vegetative cover along river banks. That change increased bank erosion and allowed widening of the river channel. Further, without firmly established riparian plants, the river channel became unstable and incised which reduced the elevation of the water. This lower channel elevation also drained valley groundwater causing drier surface soil conditions. This further reduced valley floor vegetation cover and growth. With the return of the wolves, riparian plants recovered slowly and the natural channel structure returned, groundwater elevation rose, soil moisture increased, and the more saturated soils benefited riparian vegetation growth.
Photos (Figure 4.8) illustrate the Gallatin River valley at the time the wolves were present (Figure 4.8a), the period when the wolves were eradicated (Figure 4.8b), during the long period of wolf absence (Figure 4.8c), and after wolves were reestablished as an effective predator (Figure 4.8d). This is a clear, well-documented example of a top predator having effects that go beyond herbivorous prey and plants all the way down to groundwater conditions. This case illustrates the extent to which species interactions can change ecosystem conditions.
Further effects of wolves have been documented in the Yellowstone National Park and surrounding re- gion. Coyote (Canis latrans), raven (Corvus corax), and grizzly bears (Ursus arctos horribilis) feed on elk carrion from wolf kills. This food source has improved the reproductive success and survival of these scavenger species, and changed their foraging behaviors as well (Wilmers et al. 2003). For example, grizzly bears have been known to forego hibernation altogether in Glacier National Park, Montana in favor of scavenging wolf kill sites (Wilmers et al. 2003). Without wolves, carrion availability was primarily a function of the generally severe winters when high snow levels and cold temperatures caused elk to weaken and die, usually at the end of winter. For wolves, scavenging at wolf kill sites occurs on a year-round basis. By changing the distribution and abundance of carrion availability, wolves may serve to facilitate the acquisition of food by scavengers (Wilmers et al. 2003). Wolves also decrease the year-to-year and month-to-month variation in carrion availability. By transferring the availability of carrion from the highly productive late winter to the less productive early winter, and from highly productive years to less productive ones, wolves provide a temporal subsidy to scavengers. Thus, wolves change the timing of resource availability from a pulsed resource at the end of severe winters to a more constant resource throughout the winter. This resource subsidy may in turn promote increased biodiversity and lead to larger populations of scavenger species and, in fact, studies have documented an increase in scavenger species after wolf reintroductions (Wilmers et al. 2003).

In addition, wolves may mitigate climate change effects in Yellowstone National Park. The winter period on the northern range of Yellowstone National Park has lessened since 1948 (Wilmers and Getz 2005). Evidence for winter period contraction has been seen in the form of decreased duration of snow cover, snowfall and snow depth; average temperatures increasing in late winter; and an increase in the number of winter days with temperatures above freezing (Wilmers and Getz 2005). The easing of these winter conditions implies that elk will recover sooner from the detrimental stresses of winter. Smaller snow packs allow elk easier access to food and decrease energy expenditures required for movement. Herbaceous plant growth usually begins within a few days to weeks of the last snow cover, so elk may increase the quality and quantity of food intake earlier in the year. And, elk will experience a shorter physiologically stressful winter period. These factors are likely to influence the timing and abundance of carrion as late-winter elk mortality declines. Under scenarios without wolves, scavengers could face food bottlenecks in the absence of late-winter carrion. Coyotes are highly dependent on late-winter and early-spring carrion to sustain them until late spring, when elk calves and ground squirrels become abundant. Areas without wolves will experience carrion as an increasingly pulsed resource under climate change, whereas areas with wolves will likely encounter carrion throughout the winter months. Thus wolves buffer the effects of climate change on carrion availability and allow scavengers to adapt to a changing environment over a longer time scale more commensurate with natural processes (Wilmers and Getz 2005).
In addition, increased riparian vegetation, an indirect result of wolf reintroductions, has supported a greater diversity and abundance of birds (Anderson 2007). Before the reintroduction of wolves, elk re- duced willow structure (much less cover < 2 m height) and reduced the numbers of all bird species (Anderson 2007). The least sensitive species were the habitat generalists and ground nesting birds. Willow and aspen communities support a greater diversity of flora and fauna than most other habitats in the western United States; such an enhancement of biomass can subsequently increase bird diversity and abundances in the region.
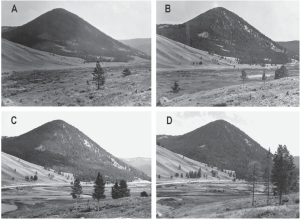
Overall, biodiversity and productivity have increased in Yellowstone National Park with wolves reestablished as a top predator in the ecosystem. The extirpation of wolves had a cascading effect on lower trophic levels (first elk and then willows) along the Gallatin River. Even though the long-term trend in elk numbers was one of decline due to the annual harvest of elk via hunting, predation by other large carnivores, and the periodic occurrence of mortality during severe winters, this situation was unable to prevent the continued decimation of streamside vegetation. The heavy annual browsing of willow communities after the loss of wolves ultimately generated major changes in floodplain functions and channel morphology. This case is the first to connect a large, highly interacting carnivore to the characteristics of a river floodplain and its channel. These findings are consistent with the rewilding argument for reestablishing a natural ecosystem by assembling a full complement of species that can reinvigorate ecological processes.
SUMMARY
The case of wolves in Yellowstone National Park was not considered a rewilding case when it started, but it is consistent with the approach and demonstrates the anticipated benefits of the hypothesis. Among conservationists and managers, the rewilding approach is controversial and subsequently, is not commonly advocated. The primary issues and concerns with rewilding focus on the introduction of non-native species, the risk of unintended results that may adversely affect the ecosystem that is being recovered, and the accompanying high visibility with the public whose support is crucial for conservation programs in general. A great deal of conservation planning emphasizes conserving existing processes rather than restoring extinct species interactions. Rewilding centers on restoring ecological function which is an optimistic goal. As more cases and studies show positive results from rewilding projects, increased acceptance will fuel further utilization of this strategy in future conservation efforts.
REFERENCES
Alladale Wilderness Reserve, 2021. Alladale Wilderness Reserve. Available http://www.alladale.com/ (September 2021).
Anderson, E.M., 2007. Changes in bird communities and willow habitats associated with fed elk. The Wilson Journal of Ornithology, 119(3), pp.400-409.
Asner, G.P., Levick, S.R., Kennedy-Bowdoin, T., Knapp, D.E., Emerson, R., Jacobson, J., Colgan, M.S. and Martin, R.E., 2009. Large-scale impacts of herbivores on the structural diversity of African savannas. Proceedings of the National Academy of Sciences, 106(12), pp.4947-4952.
Banks, P.B. and Hochuli, D.F., 2017. Extinction, de-extinction and conservation: A dangerous mix of ideas. Australian zoologist, 38(3), pp.390-394.
Beschta, R.L. and Ripple, W.J., 2006. River channel dynamics following extirpation of wolves in northwestern Yellowstone National Park, USA. Earth Surface Processes and Landforms: The Journal of the British Geomorphological Research Group, 31(12), pp.1525-1539.
Beschta, R.L. and Ripple, W.J., 2010. Recovering riparian plant communities with wolves in northern Yellowstone, USA. Restoration Ecology, 18(3), pp.380-389.
Carpenter, S.R., Kitchell, J.F. and Hodgson, J.R., 1985. Cascading trophic interactions and lake productivity. BioScience, 35(10), pp.634-639.
Donlan, C. J., Greene, H. W., Berger, J. , Bock, C. E., Bock, J. H., Burney, D. A., Estes, J. A., Foreman, D., Martin, P. S., Roemer, G. W., Smith, F. A. and Soulé, M. E., 2005. Re-wilding north America. Nature, 436(7053), pp.913-914.
Donlan, C. J., Berger, J., Bock, C.E., Bock, J.H., Burney, D.A., Estes, J.A., Foreman, D., Martin, P.S., Roemer, G.W., Smith, F.A. and Soulé, M.E., 2006. Pleistocene rewilding: An optimistic agenda for twenty-first century conservation. The American Naturalist, 168(5), pp.660-681.
Donlan, C.J., and Greene, H.W., 2010. NLIMBY: No lions in my backyard. Pages 295-307 in M. Hall (editor). Restoration and History: The Search for a Usable Environmental Past. Routledge, New York, NY.
Elton, C., 1927. Animal Ecology. Sidgwick & Jackson, Ltd., London.
Estes, J.A., Tinker, M.T., Williams, T.M. and Doak, D.F., 1998. Killer whale predation on sea otters linking oceanic and nearshore ecosystems. science, 282(5388), pp.473-476.
Fortin, D., Beyer, H.L., Boyce, M.S., Smith, D.W., Duchesne, T. and Mao, J.S., 2005. Wolves influence elk movements: Behavior shapes a trophic cascade in Yellowstone National Park. Ecology, 86(5), pp.1320-1330.
Griffiths, C.J., Jones, C.G., Hansen, D.M., Puttoo, M., Tatayah, R.V., Müller, C.B. and Harris, S., 2010. The use of extant nonindigenous tortoises as a restoration tool to replace extinct ecosystem engineers. Restoration Ecology, 18(1), pp.1-7.
Hairston, N.G., Smith, F.E. and Slobodkin, L.B., 1960. Community structure, population control, and competition. The american naturalist, 94(879), pp.421-425.
Ismail, K., Kamal, K., Plath, M. and Wronski, T., 2011. Effects of an exceptional drought on daily activity patterns, reproductive behaviour, and reproductive success of reintroduced Arabian oryx (Oryx leucoryx). Journal of Arid Environments, 75(2), pp.125-131.
Johnson, C.N., Isaac, J.L. and Fisher, D.O., 2007. Rarity of a top predator triggers continent-wide collapse of mammal prey: Dingoes and marsupials in Australia. Proceedings of the Royal Society B: Biological Sciences, 274(1608), pp.341-346.
Lodge, D.M., Kershner, M.W., Aloi, J.E. and Covich, A.P., 1994. Effects of an omnivorous crayfish (Orconectes rusticus) on a freshwater littoral food web. Ecology, 75(5), pp.1265-1281.
Marris, E., 2009. Conservation biology: Reflecting the past. Nature News, 462(7269), pp.30-32. Martin, P.S., 2005. Twilight of the mammoths: Ice Age extinctions and the rewilding of America (Vol. 8). Univ of California Press.
Novak, B.J., 2018. De-extinction. Genes, 9(11), p.548.
Pace, M.L., Cole, J.J., Carpenter, S.R. and Kitchell, J.F., 1999. Trophic cascades revealed in diverse ecosystems. Trends in ecology & evolution, 14(12), pp.483-488.
Paine, R.T., 1969. A note on trophic complexity and community stability. The American Naturalist, 103(929), pp.91-93.
Paine, R.T., 1980. Food webs: Linkage, interaction strength and community infrastructure. Journal of animal ecology, 49(3), pp.667-685.
Paine, R.T. and Schindler, D.E., 2002. Ecological pork: Novel resources and the trophic reorganization of an ecosystem. Proceedings of the National Academy of Sciences, 99(2), pp.554-555.
Parker, K.A., Seabrook‐Davison, M. and Ewen, J.G., 2010. Opportunities for nonnative ecological replacements in ecosystem restoration. Restoration Ecology, 18(3), pp.269-273.
Pettersson, H.L. and de Carvalho, S.H.C., 2021. Rewilding and gazetting the Iberá National Park: Using an asset approach to evaluate project success. Conservation Science and Practice, 3(5), p.e258.
Ripple, W.J. and Beschta, R.L., 2007. Restoring Yellowstone’s aspen with wolves. Biological Conservation, 138(3-4), pp.514-519.
Ripple, W.J. and Beschta, R.L., 2012. Trophic cascades in Yellowstone: The first 15 years after wolf reintroduction. Biological Conservation, 145(1), pp.205-213.
Rubenstein, D.R., Rubenstein, D.I., Sherman, P.W. and Gavin, T.A., 2006. Pleistocene Park: Does re- wilding North America represent sound conservation for the 21st century? Biological Conservation, 132(2), pp.232-238.
Sandom, C., Wynne-Jones, S., Pettorelli, N., Durant, S. and Du Toit, J., 2019. Rewilding a country: Britain as a study case. Rewilding, pp.222-247.
Schmitz, O.J., Hambäck, P.A. and Beckerman, A.P., 2000. Trophic cascades in terrestrial systems: A review of the effects of carnivore removals on plants. The American Naturalist, 155(2), pp.141-153.
Schmitz, O.J., Hawlena, D. and Trussell, G.C., 2010. Predator control of ecosystem nutrient dynamics. Ecology letters, 13(10), pp.1199-1209.
Segar, J., Pereira, H.M., Filgueiras, R., Karamanlidis, A.A., Saavedra, D. and Fernández, N., 2021. Expert‐based assessment of rewilding indicates progress at site‐level, yet challenges for upscaling. Ecography.
Sergio, F., Caro, T., Brown, D., Clucas, B., Hunter, J., Ketchum, J., McHugh, K. and Hiraldo, F., 2008. Top predators as conservation tools: Ecological rationale, assumptions, and efficacy. Annual review of ecology, evolution, and systematics, 39, pp.1-19.
Shapiro, J. and Wright, D.I., 1984. Lake restoration by biomanipulation: Round Lake, Minnesota, the first two years. Freshwater biology, 14(4), pp.371-383.
Sutherland, W.J., 2002. Openness in management. Nature, 418(6900), pp.834-835.
Taylor, H.R., Nelson, N.J. and Ramstad, K.M., 2019. The first recorded interaction between two species separated for centuries suggests they were ecological competitors. New Zealand Journal of Ecology, 43(1), pp.1-6.
Terborgh, J., Lopez, L., Nuñez, P., Rao, M., Shahabuddin, G., Orihuela, G., Riveros, M., Ascanio, R., Adler, G.H., Lambert, T.D. and Balbas, L., 2001. Ecological meltdown in predator-free forest fragments. Science, 294(5548), pp.1923-1926.
Vera, F.W., 2009. Large-scale nature development–The Oostvaardersplassen. British Wildlife, 20(5), p.28.
Wallach, A.D., Johnson, C.N., Ritchie, E.G. and O’Neill, A.J., 2010. Predator control promotes invasive dominated ecological states. Ecology letters, 13(8), pp.1008-1018.
Wilmers, C.C., Crabtree, R.L., Smith, D.W., Murphy, K.M. and Getz, W.M., 2003. Trophic facilitation by introduced top predators: Grey wolf subsidies to scavengers in Yellowstone National Park. Journal of Animal Ecology, 72(6), pp.909-916.
Wilmers, C.C. and Getz, W.M., 2005. Gray wolves as climate change buffers in Yellowstone. PLoS biology, 3(4), p.e92.